Professor Steven Prawer from the School of Physics at the University of Melbourne, wants everybody to have diamonds. To improve their quality of life, of course.
He’s not imagining diamond rings or jewellery; he’s envisaging quantum computers and health monitors made of diamond.
He wants to harness the same clarity and purity that make diamonds sought-after for jewellery. But in his vision that clarity will efficiently guide lasers, measure the faint flashes of a quantum calculation, or sift out individual strands of cancer DNA from a drop of blood.
“It’s got lovely properties – you can see a single atom shine brightly in a diamond and tell what the spin is.
“You can manipulate its spin and do it all at room temperature. You don’t need to cool it down like silicon,” Professor Prawer says.
Room-temperature operation is a major boon in the race for dominance in the quantum computing industry, which promises to be a multi-billion-dollar concern.
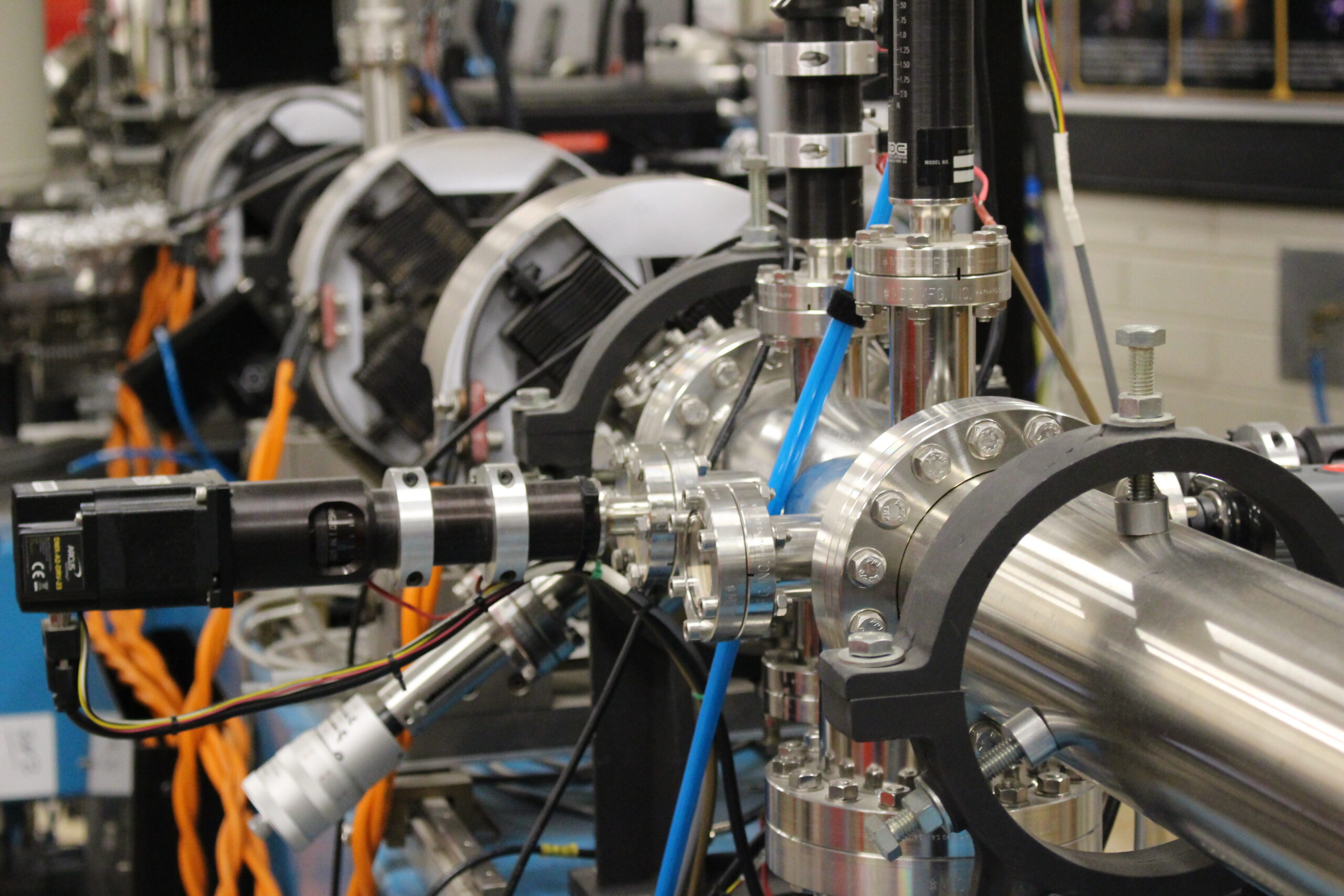
And diamond could be an important in another billion-dollar industry. With the cost of healthcare skyrocketing, Professor Prawer says serious, long-term investment in research into personalised, take-home, early-detection technology is no-brainer.
But results won’t come overnight: Professor Prawer and his team have spent more than a decade at the Heavy Ion Accelerators lab of the Melbourne University node, honing techniques to create the perfectly flat layers of pure diamond they can produce today.
The biggest hurdle they have had to overcome has been one of diamond’s most famed qualities – its hardness.
Although great for durability and corrosion resistance, that hardness makes the technology for processing diamond very challenging. The silicon industry uses chemical etching to shape components from templates, but there is no chemical that can etch diamond.
Instead, Professor Prawer found the best way to carve shapes in diamond was by drawing shapes with ion implantation, with the Pelletron accelerator at the University of Melbourne. Where the ions bombard the diamond their energy converts the diamond to graphite, which can be etched off with strong acids, leaving clean nanostructures of diamond.
These structures can take the form of an extremely thin layer, engineered to have a precise and very small thickness. Such exactness enables light to be trapped and steered around inside the diamond layer to connect into the quantum properties of its optical centres.
To carve layers this thin leverages on a trick of physics, in which ions pass through the upper parts of the diamond causing only minimal damage, and dump most of their energy in a small volume as they grind to a halt.
However, initial experiments with this engineered top layer were disappointing.
“We made all kinds of interesting structures that looked absolutely terrific, such as the world’s smallest diamond ring , but it turned out there was too much damage in them, they weren’t quantum active,” Professor Prawer says.
“The minor damage caused by the ions on the way in can be annealed, but not enough to restore the quantum activity.”
All was not lost, however. The team realised they could use the crippled layer as a template, and grow a thin layer of new, pure diamond on top of it. To complete the process, they flipped it over and used another ion bombardment technique, reactive ion etching, to remove the template and expose the pure newly grown diamond layer.
The result is an incredibly smooth layer, of dimensions up to 7 by 7 millimetres, and as thin as 250 nanometres – less than a wavelength of the light emitted by diamond nitrogen-vacancy quantum centres. Because it has been grown, the layer is not strained, and sits flat without rolling up.
The team then finessed the process, attaching handles to pick up and manipulate the samples, and making myriad shapes, and even structures hanging over edges. They also used a mosaic of large diamond seeds to create multiple copies, demonstrating that scaling up to industrial volumes is possible.
Such smooth layers are a crucial component for a room-temperature quantum computer. The nitrogen vacancy (NV) centre qubit at the heart of a diamond quantum computer operates on flashes of light as it moves between different spin states. Professor Prawer’s diamond layers are the analogue of the metal and semiconductor electronic components that sit on a silicon chip around a silicon qubit.
“It’s the missing element of the technology road map, how to capture the light and route it around,” Professor Prawer says.
“This ion beam gives us an essential part of the diamond nano-fabrication toolkit.”
With the ability to control light in diamond sewn up, Professor Prawer is thinking of more sophisticated biological applications.
Tiny pores through a diamond layer could be used as sensors if they match the size of individual molecules produced by diseases, which might be in a drop of bodily fluid. Some approaches use electrical charge, but Professor Prawer says diamond’s optical properties have potential.
“You could detect the passage of a molecule through the pore with luminescence. The diamond NV centre is very sensitive – a magnetically tagged molecule would see a large signal.
“We have tests for covid, what more could we do?” he asks.
“A grand challenge for the entire population’s health would be early screening for cancer. If we’re going to improve quality of care cost-effectively, we need to bring in high technology. We need to think a little differently!”
HIA Featured Experts and Facilities:
- Professor Jeffrey McCallum, University of Melbourne, Experimental Condensed Matter Physics Laboratory.